Challenges of Removing Fine Iron from Powders
August 28, 2017
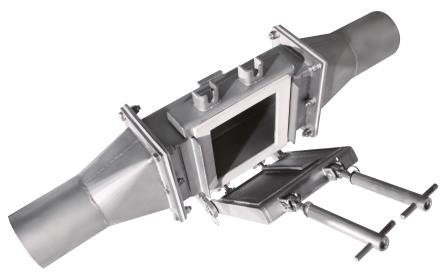
Removing metal contamination when the tramp metal and material is granular is far more straightforward than when in a powder form. To determine the best solution to remove fine iron contamination from powders, it is necessary to have a good understanding of the way the fine materials behave.
Powders are produced and used in a wide variety of industries including food, pharmaceuticals, and chemicals. It is estimated that 80% of materials used in industry are in a powdered form. A “powder” is defined as fine dry particles produced by the grinding, crushing, or disintegration of a solid substance. The nature of a powder means that the handling and processing tends to be problematic as powders exhibit similar properties to both solids and liquids.
Metal contamination, commonly in an iron form, can be introduced into a material at any stage within a process. Tramp metal that is undetected and remains in the product before the powder production stage, becomes significantly reduced in size and, subsequently, increasingly difficult to extract.
Magnetically susceptible metal contamination (i.e. iron) is commonly removed using magnetic separators. There is magnetic separation equipment that traps metal using ceramic or neodymium iron boron (neodymium). Ceramic magnets produce low strength but deep reach magnetics fields, while neodymium magnets create the strongest permanent magnetic products presently commercially available.
Where Does the Metal Originate?
Metal contamination commonly originates in a powder from two sources: Primary large tramp metal, such as a nail, screw, or bolt; primary or secondary fine tramp iron. Primary fine iron or magnetic particles are often present in the raw material. This originates from primary processing, transportation, or even naturally occurs in the original material. Secondary fine iron originates from a larger tramp metal source that has been reduced in size during the process. Typically, this could be from a nail, screw, or bolt that has been through a size reduction process, or from damaged or worn processing equipment. Another common source of secondary fine iron contamination is rust falling into the process from weathered and worn processing equipment such as chains, hoists, and building cladding.
The separation and detection of tramp metal is easier when the metal contamination is in a larger form and can be successfully removed using a wide range of suitable magnetic separators and metal detectors. Magnetic separators using standard strength ceramic magnets, with deep magnetic fields, are ideal. A good example is the plate magnet, often installed in a chute, in a housing, or as part of an in-line magnetic separator.
Larger metal contamination is also easier to detect on a metal detector. Metal is detected as it passes through the coil of the metal detector and an automatic reject system removes it from the flow. For detection, the magnetic field generated by the metal detector has to see a state change. Finer sized metal produces a lesser state change and thus increases the difficulty in detection.
Removing larger tramp metal with a magnetic separator and metal detector prior to the processing stage not only prevents the metal from being reduced in size (e.g. converted into a secondary source of fine iron contamination), but also protects delicate processing equipment such as granulators, shredders, and mills from being damaged by the metal.
Once in a powder form, there are processing parameters to consider when assessing the optimum method to remove fine iron contamination.
How Does a Powder Flow?
When a powder is sprinkled, it remains light and free. However, when the same powder is vibrated or compressed, it may become very dense and even lose the ability to flow.
Individual grains in a powder cling to each other in clumps, in accordance with the Van der Waals force. This coagulation often results in the fine iron being trapped in among clean product. The ability of any magnetic separator to attract, hold and separate the fine iron is dependent on the iron being as close to the magnetic field as is physically possible. If the fine iron contacts the surface of a magnetic separator with a high-strength magnetic field, it will be held. However, when the fine iron is held inside a coagulation of powder, then it could be held out of the reach of the maximum magnetic force. Thus, it will not be separated.
The way a powder flows impacts on the design of the magnetic separator. Powders flowing in a hopper may experience classic flow problems such as bridging or flooding, all of which could be exacerbated by the design of magnetic separator.
Different Designs of Magnetic Separators
High-strength magnetic fields, as produced by neodymium, are needed to capture fine iron metal contamination. These are the main magnet configurations suitable for handling powders:
a. Tubular magnetic cartridges, often in a multi-rod grate configuration
b. Flat-faced magnetic plates
c. Cone-shaped magnets
d. Magnetic drums with a curved magnetic arc
Although occasionally a magnetic cartridge may be used on its own, it is more commonly part of a larger multi-cartridge grate system. The magnetic grate is designed to fit inside a hopper, or can be supplied complete with a housing (i.e. as a drawer filter magnet).
In operation, powder falls freely onto the surface of the magnetic cartridge where fine iron strikes the surface and is held by the strong magnetic field. To ensure that the powder makes contact with the cartridge surface, deflectors are often deployed above the gaps between the cartridges.
Powder build-up on the surface of a magnetic cartridge will reduce the separation efficiency. Also, in severe cases, a slight build up on the surface of the cartridge may quickly cause a blockage of the whole housing.
Such blockages can be prevented by ensuring that there is optimum space between the magnetic cartridges. Also, in some cases, the mounting of an external vibrating motor on the side of the hopper or housing will provide enough disturbance to prevent any material coagulation. The frequency of the vibration needs careful consideration as it could affect the flow ability of the powder. Additionally, when vibrators are used, the magnetic cartridges need to be manufactured to withstand prolonged periods of vibration.
Flat-faced magnetic plates are ideal when it is possible for the material to flow over the surface. For fine iron removal, the magnetic plates would use high strength neodymium magnets. This magnetic field is further enhanced when a tapered step is added to the face of the magnet. Captured iron migrates behind the step and away from the material flow, reducing the risk of re-entering the cleansed product.
As well as being fitted into chutes, magnetic plates are incorporated into housings. The plate housing magnets resist bridging and choking to remove tramp iron and ferrous fines from flow-resistant bulk materials. The stainless steel housings mount easily to enclosed spouting or directly on processing equipment.
There are optional square, rectangular, and round adapters for easy connection to existing chute work. A baffle at the top of the housing helps break up clumps and directs product flow over the unit’s two powerful plate magnets.
Plate magnets are also used in in-line magnets and there are two designs:
1. Gravity in-line magnets: The plate magnets are positioned in round, sloping spouting where material is under gravity flow. For effective tramp metal capture, the sprouting should be angled no more than 60° from horizontal
2. Pneumatic in-line magnets: These designs are for use in dilute phase pneumatic conveying systems (up to 15 psi). They can be installed easily with optional factory-supplied compression couplings and work best in horizontal runs with the plate magnet down to take advantage of material stratification
Another design of in-line magnet is the center-flow, although the magnetic field is generated in a Cone configuration instead of a plate. The magnetic cone is positioned in the center of the housing, allowing the powder to flow in the space left between the housing. Center-flow in-line magnetic separators are commonly used in dilute-phase pneumatic conveying lines up to 15 psi.
To achieve optimum contact with the product flow, a conical magnet is suspended in the center-line of the housing. This tapered, exposed-pole cartridge has a stainless steel “nose cone” to direct the flow of materials around the magnet. The tapered poles of the cone magnet allow ferrous fines to collect out of the direct air stream. Additionally, the trailing end of the magnet is an active magnetic pole and holds any tramp metal that is swept down the cone.
Both types of in-line magnets are designed with clamps and doors to enable easy access for cleaning.
In specific applications, a high-strength neodymium drum magnet will enable the best level of separation. The drum magnet is gravity-fed, usually via a vibratory feeder. The drum magnet has a stationary high-strength magnetic arc positioned inside a rotating outer shell. When material flows onto the drum magnet, the magnetic field projected by the stationary magnetic assembly inside the shell captures fine iron and holds it securely to the drum’s stainless steel surface. With contaminants removed, the good product falls freely to a discharge point. As the drum rotates, the captured fine iron travels along the drum surface and out of the magnetic field, where it is discharged.
There are various magnetic field configurations possible, but the most suitable for removing iron from powder is one that produces a radial magnetic field. This ensures that once captured, the fine iron does not leave the drum surface until it moves out of the magnetic field.
Processing powder on a drum magnet presents more difficulties that other designs of magnetic separators. First, it is recommended that the vibratory feeder has an air bed to produce a consistent feed of powder. Standard vibratory feeders may deliver powder in clumps, significantly affecting the separation performance.
Second, the shell of the drum magnet should be rotated at high speeds. This will result in some of the powder pluming, and this can be minimized by keeping the distance between the end of the vibratory feeder tray and the rotating surface of the drum magnet to a minimum.
The high rotation speed of the drum magnet significantly reduces the amount of product lost to the magnetics. This is because there is less material on the surface of the drum at any one time, reducing the chance of entrapment.
The use of drum magnets operating at high rotational speeds has been successful in removing fine iron from abrasives, refractories, and other applications where the material has a high specific gravity.
As the demand for finer and purer powders increases, so does the need to remove even the finest iron. Understanding the properties and behavior of the powder is vitally important when considering the optimum method of fine iron separation. Often the ultimate solution is a series of magnetic separators and metal detectors located at strategic points within the process.
Brock Herrmann is product manager, metal separation, Bunting Magnetics Co. For more information, visit buntingmagnetics.com.
For related articles, news, and equipment reviews, visit our Screening & Separation Equipment Zone
Click here for a List of Screening & Separation Equipment
You May Also Like